Effects of curvature and composition on membrane structure and dynamics
We have recently developed tools for the study of lipid diffusion on curved surfaces, including those with Gaussian curvature. These tools were also used to study how Gaussian curvature sorts lipid phases and alters their diffusion (pictured above). We have also demonstrated how membrane curvature can lead to anisotropic diffusion. Currently, we are studying how membrane curvature and lipid chemistry play roles in dictating whether phase-separated membranes adapt a registered or anti-registered conformation (pictured below). This work is done in close collaboration with Dr. Balázs Fábián (MPI Biophysics).
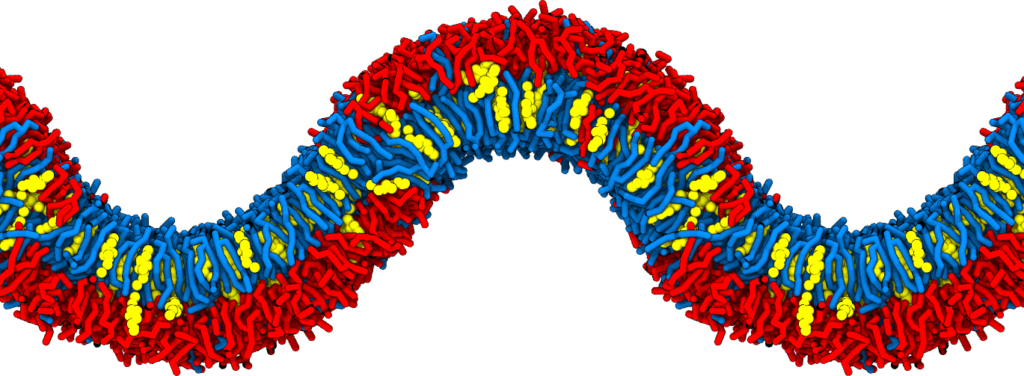
We have recently also inspected the nature of the liquid ordered phase at different temperatures together with experimentalists, and evaluated the effects of lipid composition, temperature, and protein concentration on the viscosity of lipid membranes. Currently, we are trying to understand the differences in the dynamics of lipid bilayers and monolayers using both experiments and simulations with Prof. Salvatore Chiantia (University of Potsdam).
Structure and function of the Sec61/TRAP translocon
Based on a cryo-EM structure, we used simulations to unravel the potential role of the translocon-associated protein (TRAP)—a key partner of the Sec61 translocon—in the translocation of certain challenging substrates. We also performed simulations to help understand the binding mode of a Sec61 inhibitor. Most recently, we described the role of Sec61/TRAP complex as a potential lipid scramblase, and characterised its scrambling activity in detail. Most of this work was performed during my time as a postdoc in the group of Dr. Ville Paavilainen (Institute of Biotechnology, University of Helsinki).

Simulation model for the pulmonary surfactant
We have demonstrated and later confirmed that with a better water model, simulations with atomistic force fields can reproduce experimental data for single-component monolayers, also in the presence of ions. These findings motivated us to build a model for the pulmonary surfactant. We first included a realistic lipid composition in the model, followed by the incorporation of hydrophobic surfactant proteins. Most recently we have used the model to study the effects of electronic cigarette flavouring chemicals on lung surfactant structure and function. This work is performed in close collaboration with Prof. Lukasz Cwiklik (J.Heyrovský Institute of Physical Chemistry of the Czech Academy of Sciences), Prof. Ilpo Vattulainen (University of Helsinki), and Dr. Jorge Bernardino de la Serna (Imperial College).

Highlights of recent model and method development
As a part of the group of Prof. Pavel Jungwirth, I was involved in the development of a charge-scaled biomolecular force field that would capture electronic polarisation without an additional simulation cost. After years of hard work, we recently published the product, the prosECCo75 force field.
We have also been involved in the NMRlipids project led by Dr. Samuli Ollila (Technical Research Center of Finland). Most recently, we benchmarked the ability of atomistic force field to describe the structural and dynamic effects of cholesterol on lipid bilayers.
Recent methodological work also involves the development of a membrane simulation model containing a thickness gradient (pictured) for the study of hydrophobic mismatch -driven lateral sorting of, e.g., peptides, and the development of a method to simulate asymmetric solvent conditions across membranes.