Our goal at the experimental quantum optics group is to make photons obey our whims. We develop methods for shaping their spatio-temporal structure, interfere them with other bosons, and have them interact with novel structures and materials. However, we do not limit ourselves to single photons, and sometimes venture out to the world of classical states of light.
For a more in-depth description of what this means,
-> here you can find descriptions of how we structure light in all of its degrees of freedom and multiple tasks for which these structuring methods are useful
-> here you can read more about how we use these methods for high-dimensional quantum information
-> here you can dive into how we use nonlinear optics and novel materials to study and harness light-matter interactions on a single photon level, and how we explore the nature of plasmons
… and here, you can find out with whom we are collaborating on these research endeavors!
Complex structuring of light
Structuring light in the temporal and spatial domains has led to a myriad of fascinating research findings, in both the classical and quantum realms and to countless beautiful applications. These findings and applications range from advanced interactions with atoms, molecules, and macroscopic matter to insights into the fundamental nature of light fields and novel quantum states. One aspect of our research efforts is to build and expand on this previous work, e.g., through developing fruitful applications for complex structured light and, more importantly, pushing the field forward by constructing increasingly complex light fields through a creative combination of all photonic degrees of freedom.
Amongst other things, we are working on developing and applying self-accelerating beams in the spatial domain. A recent example is the application of radially self-accelerating beams, i.e., light fields that spiral around the optical axis (see Fig. 1), for measuring longitudinal positions in a simple and highly accurate manner [1]. Our research on self-accelerating beams focuses on their intrinsic challenges and limitations, in laboratory implementations, thereby laying the foundations for future applications.
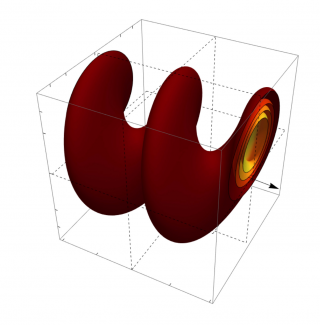
In the EQO group, we also study the interplay between different degrees of freedom in the context of structured light. Recent history has demonstrated that combining two degrees of freedom, for example, polarization and the transverse spatial domain, leads to many beautiful effects and applications in quantum simulation and communication tasks. Building on these findings, we are studying methods for extending these ideas to other domains, such as the spectral domain.
One recent study has led to a novel spectroscopy technique, which benefits from a strong correlation between the frequency components and the polarization state of a broadband light field (see sketch in Fig. 2). A beam with a frequency-dependent polarization enables a high-speed read-out of spectroscopic measurements through fast polarization analysis [2]. In future studies, we intend to further increase the complexity of the field by also including the spatial degrees of freedom. We will also investigate such states in the context of quantum experiments, i.e., test their properties in connection to features such as the quantum entanglement of multiple photons.
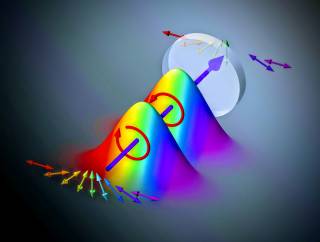
In case you want to learn more about structured light including all degrees of freedom there is a recent roadmap that might interest you [3].
High-dimensional quantum photonics
Together with the more general studies on structured light and the development of advanced tools (described here), in the EQO group, we investigate complex structured single photons (see Fig. 3) as a physical realization of a high-dimensional quantum state. High-dimensional quantum information science is a very promising field where multiple examples of its superiority to two-level systems, i.e., qubits, have been shown [4]. However to some extent, it is still in its infancy.

To fully utilize the power of high-dimensional quantum states and test their limitations, the development of advanced tools for transforming photons, into and within complex structures, is required. Recently, we have demonstrated the applicability and prospects of using a multi-plane light conversion (MPLC) scheme to manipulate spatial structures in the quantum domain. In this scheme, the spatial extent of the beam is modulated with various consecutive modulation planes (see Fig. 4). Upon propagating through these planes, a complete and, in principle, lossless modulation of the beam shape is achieved. Using this MPLC scheme, we have shown that it can perform a near-perfect projection of high-dimensional quantum states encoded on photons [5], it can be used to realize any high-dimensional quantum gate [6], and it can be used to construct a flexible linear optical network along a single beamline [7].
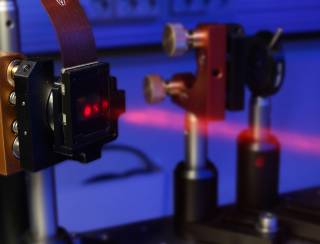
Our current research efforts involve but are not limited to, studying the application of these ideas for generating super-sensitive quantum states of light. We are also developing ways to realize integrated solutions for MPLC. Moreover, we are investigating how to invoke other photonic degrees of freedom, such as the polarization and spectral/temporal domains. Combing multiple degrees of freedom will dramatically increase the state space dimensionality of a single photon, thereby enriching the field of high-dimensional quantum information science.
Light-matter interactions on the quantum level
In the EQO group, another important research theme is the interaction of light with matter, with a particular focus on the quantum domain. Here, one emphasis is on the study and optimization of one of the most important workhorses for photonics experiments, the nonlinear optical effect of spontaneous parametric down-conversion (SPDC). Together with some of our collaborators, we are investigating the strong nonlinearities of nanostructured metasurfaces, ENZ-materials, and 2D-materials with a focus on their use in the quantum optics domain.
Additionally, using the nanofabrication facilities and in-house expertise at Tampere University, we are studying the interplay between quantum photonics and quantum plasmonics. Ongoing research efforts include the detailed study of the possible interference of both single photons and entangled photons pairs with surface plasmon polaritons (SPP) generated via plasmonic nanostructures.
Collaborators
In-house:
> Regina Gumenyuk and her fiber laser group
> Mikko Huttunen and his nonlinear optics group
> Tapio Niemi and his nanophotonics group
> Marco Ornigotti and his theoretical optics group
National:
> Zhipei Sun and his photonics group (Aalto University)
International:
> Frederic Bouchard (National Research Council, CA)
> Bob Boyd and his research group (University of Ottawa, CA)
> Yuri Chamorovskii and his research group (Kotelnikov Institute of Radio Engineering and Electronics, RU)
> Israel De Leon and his group (Tecnológico de Monterrey, MX)
> Enno Giese and his group (TU Darmstadt , DE)
> Marcus Huber and his research group (TU Vienna, AT)
> Sven Ramelow and his research group (Humboldt University, DE)
> Benjamin Stickler (University of Duisburg-Essen, DE)
> Abuzer Yakaryilmaz (University of Latvia, LV),
References:
[1] S. Prabhakar, S. Plachta, M. Ornigotti, R. Fickler, High-accuracy longitudinal position measurement using self-accelerating light, Applied Optics 60 (11), 3203 (2021) link
[2] M. Piccardo, V. Ginis, A. Forbes, M. Hiekkamäki, R. Fickler, et al. Roadmap on multimode light shaping, arXiv:2104.03550 (2021) link
[3] L. Kopf, J. R. Deop Ruano, M. Hiekkamäki, T. Stolt, M. J. Huttunen, F. Bouchard, R. Fickler, Spectral vector beams for high-speed spectroscopic measurements, Optica 8, 930 (2021) link
[4] M. Erhard, R. Fickler, M. Krenn, A. Zeilinger, Twisted Photons: New Quantum Perspectives in High Dimensions, Nature Light: Science & Applications, 7 17146 (2018) link
[5] M. Hiekkamäki, S. Prabhakar, R. Fickler, Near-perfect measuring of full-field transverse-spatial modes of light, Optics Express 27 (22), 31456 (2019) link
[6] F. Brandt, M. Hiekkamäki, F. Bouchard, M. Huber, R. Fickler, High-dimensional quantum gates using full-field spatial modes of photons, Optica 7, 98 (2020) link
[7] M. Hiekkamäki, R. Fickler, High-Dimensional Two-Photon Interference Effects in Spatial Modes, Phys. Rev. Lett. 126, 123601 (2021) link