Nonlinear microscopy
Nonlinear microscopy using cylindrical vector beams
Nonlinear optical effects are significantly influenced by polarized light. We further boost the sensitivity of nonlinera microscopy techniques by exploiting the polarization structure of beams with inhomogeneous states of polarization such as cylindrical vector beams (Bautista, 2012). The two most common examples of cylindrical vector beams include radial and azimuthal polarizations. When radial polarization is tightly focused, a significant longitudinal electric field component is generated along the axis of beam propagation. Tightly focused azimuthal polarization maintains strictly transversal electric fields at the focal volume. We have shown that the unique focusing properties of these beams can be used to efficiently couple light to various types of nanostructures (e.g., single or their microscopic assemblies) and to characterize such nanostructures in a new perspective.
Experimental synthesis and characterization of engineered focal fields
Significant advances in the fabrication of new kinds of photonics nanostructures promote simultaneously the increasing demand for new kinds light fields to investigate the linear and nonlinear optical responses of such nanostructures. Using state-of-the-art devices and instruments that modulate the phase and polarization structures of light, we were able to experimentally generate new kinds of light fields with unconventional vectorial properties at the focal volume. Coupled with advanced kinds of photonics nanostructures, we have shown that these new vector beams can be characterized well in three dimensions.
Multiphoton microscopy of biological tissues
We utilize multiphoton microscopy (MPM) to investigate biological tissues. The main advantage of multiphoton microscopy modalities is the good and intrinsic contrast, which arises from the fact that nonlinear optical processes inherently occur in many biologically relevant tissues. This facilitates label-free imaging of tissues making it considerably simpler to study them. We also combine MPM with machine learning techniques, such as deep learning, in order to provide fast and automated analysis of MPM images. Previously, we have for example studied lipid droplet composition of cells (Bautista, 2014) and murine ovarian tissues associated with high-grade serous carcinoma (Huttunen, 2018).
We are also developing label-free nonlinear super-resolution microscopy techniques, that could be utilized to investigate biological tissues without using any external markers (Huttunen, 2017). We believe, that we can achieve lateral resolution better than 100 nm, beating the well-known Abbe’s diffraction limit (~200 nm).
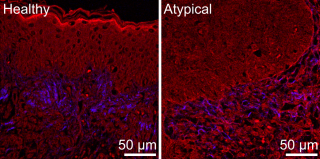
Nonlinear nanostructures and metamaterials
Design, fabrication, characterization and application of new nonlinear photonics nanostructures
The exploration of new kinds of nonlinear materials is an active research area. More importantly, there is continuous demand to increase the realizable conversion efficiencies of nonlinear effects at the single nano-object level. By exploiting the new information about the nonlinear responses from such nanostructures, we direct our efforts in improving the design, fabrication and performance of photonics nanostructures (e.g., nanoclusters, plasmonic nano-objects, semiconductor nanodisks and nanowires, nanocrystals, and even their microscopic assemblies).
Plasmonic metasurfaces
Optical responses of metals are dictated by the movement of their conduction electrons. Interestingly, in metal nanoparticles this movement exhibits resonance behavior, giving rise to localized surface plasmon resonances (LSPRs). When nanoparticles are excited near these resonant wavelengths, the local optical field near the nanoparticles is greatly enhanced resulting in strong light-matter interactions. Because nonlinear optical processes depend on the higher powers of the local field, they are enhanced even more strongly (Kauranen, 2012). Recently, it has been realized that when nanoparticles are arranged periodically, they can start behaving collectively resulting in resonances with very narrow linewidths. Importantly, these collective resonances, known as surface lattice resonances (SLRs), can further enhance the local field and further boost the nonlinear responses (Huttunen, 2016 & 2018). We are currently investigating how these collective resonance could be utilized to realize nonlinear plasmonic metamaterials that could rival conventional nonlinear materials (Czaplicki, 2018).
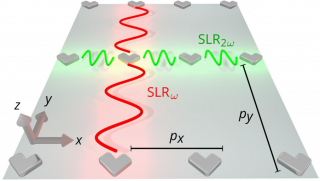
Dielectrics and two-dimensional materials
In addition to investigating metals, we also study the nonlinear responses of dielectrics(and semiconducting) materials. The intrinscic nonlinearities of dielectric materials are generally orders of magnitude weaker than that of metals. However, some of the high-refractive-index materials, such as silicon nitride (Ning, 2012) or molybdenum disulfide thin films (Karvonen, 2017), exhibit surprisingly strong nonlinear responses. In addition, dielectric materials are often dramatically less lossy than metals, allowing to fabricate resonant structures (such as gratings or ring resonators) associated with very strong field enhancements and therefore dramatically boosted nonlinear responses.
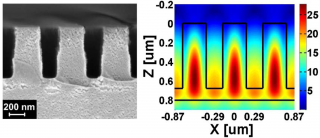
Microscopic origins of nonlinear response
Surface vs. bulk response
The birth of nonlinear optics is commonly traced to 1961 when Franken et al. used a pulsed ruby laser to demonstrate second-harmonic generation from quartz. Although a long time has passed since then, the debate over the microscopic origins of the nonlinear response persist (Wang, 2009). The particular issue is in correctly separating the surface (electric dipole origin) and bulk (magnetic dipole and electric quadrupole origin) contributions in the second-order nonlinear optical responses of materials, and in understanding their origins already at the microscopic level.
In order to answer these open and fundamental questions, we also develop nonlinear surface characterization techniques (such as two-beam approach), and use them to investigate thin films and surfaces (Koskinen, 2018).
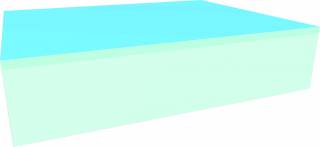
Development of numerical simulation tools
We are primarily an experimental research group aiming to investigate the interesting nonlinear physics occurring in intense light-matter interactions via series of experiments. However, we also strongly believe that development and subsequent use of accurate simulation tools is a crucial part of our work, as a good understanding of the occurring phenomena forms the basis and provides the motivation for the experimental work.
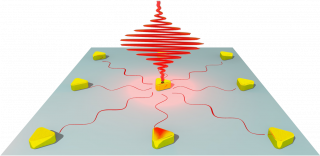
In this regard, we develop and use numerical simulation tools to understand nonlinear responses of nanoparticles (Mäkitalo, 2011). Recently, we have also been interested in improving our understanding of the collectively behaving periodic arrangements of nanoparticle arrays (Huttunen, 2018), nanoparticle oligomers (Zang, 2019) and semiconducting nanoparticles (Camacho-Morales, 2019).
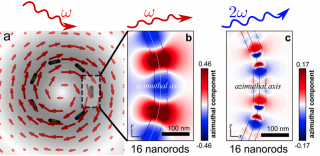