For a more in-depth description of what this means,
-> here you can find descriptions of how we structure light in all of its degrees of freedom and what we then do with such complex structured light fields
-> here you can check out how we study the interaction of structured light with matter in the linear, nonlinear, classical, and quantum optics domain
-> here you can read about our endeavors on building a coherent source of electrons and ions matter waves
… and here, you can find out with whom we are or have been collaborating on these research studies!
If you want to get an impression how our labs look, you check here.
And if you want to know which structures of light you might see in the wilderness, you might want to have a look here 😉
Complex structuring of light
Structuring light in the temporal and spatial domains has led to a myriad of fascinating research findings, in both the classical and quantum realms, and it led to countless beautiful applications. These findings and applications range from advanced interactions with atoms, molecules, and macroscopic matter to insights into the fundamental nature of light fields and novel quantum states. For various of these studies, we teamed up with our colleagues from the Theoretical Optics and Photonics group.
Multi Plane Light Conversion
One aspect of our research efforts is to develop novel methods for complex structuring of light in space, frequency, and its polarization degree of freedom. Here, a key scheme is the technique of Multi Plane Light Conversion (MPLC), which is a method that enables the performance of unitary transformations on the beam and, by that, manipulate its complex structure. The technique consists of modulating the beam profile through multiple phase modulations separated by a free space propagation, thereby allowing not only phase but also amplitude modulations (see Fig.1). We applied the method already to both the classical and quantum optics domains, e.g. through near-perfect projections of photonic high-dimensional quantum [click], the realization of high-dimensional quantum gates [click], and the construction of a flexible linear optical network along a single beamline that enables novel quantum interference effects [click]. Inspired by the resulting quantum states, we explored their application to quantum metrology [click] and investigated the behavior of the Gouy phase in the quantum realm [click]. Additionally, we have shown that an MPLC can act as an interface between multi-mode & single-mode fibers [click], coupling of free-space to waveguide modes, and to demonstrate the power of various states for advanced rotation sensing schemes [click,click]. Moreover, we are currently investigating to modulate not only the phase and amplitude but also polarization of light, pushing the capabilities of the MPLC method to the next level. Additionally, in the RCF funded project BIQOS we develop new techniques for structuring all degrees of freedom of light using laser direct writing method in an integrated way.
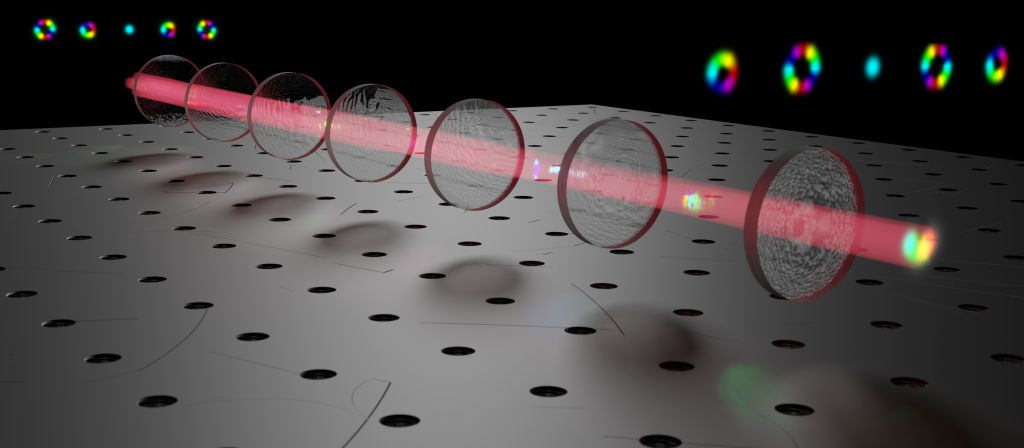
Shaping Light in all Degrees of Freedom
We also study the interplay between different degrees of freedom in the context of structured light. Recent history has demonstrated that combining multiple degrees of freedom leads to many beautiful effects and applications in classical and quantum optics. Building on these findings, we are studying methods for adapting and extending these ideas through including all degrees of freedom and investigate their peculiar properties.
After first steps to correlate the polarization degree with the frequency components of a laser pulse and study their application to high-speed spectroscopy [click], we recently went a step further and generated spatio-spectral vector beams, i.e., laser pulses that are FUlly Nonseparable in space, spectrum, and polarization. We showed that they show some similarity to tripartite entangled quantum states (GHZ states) [click] and explored their connections to higher-order Poincaré spheres [click] (see Fig. 2). Right now, we are pushing these ideas to the quantum domain, so stay tuned for complex structured entangled photons with interesting properties.
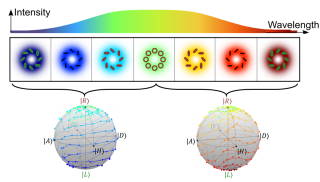
In case you want to learn more about structured light including all degrees of freedom there is a recent roadmap that might interest you [12].
Talbot effect in cylindrical systems
Together with our collaborators (e.g. our in-house fiber laser experts of the Advanced Coherent Sources group) we have investigated the Talbot self-imaging effect in the azimuthal angle and orbital angular (OAM) degrees of freedom. The self-imaging effect occurs when a periodic field in one domain acquires a quadratic phase in the corresponding Fourier domain. For azimuthal self-imaging, the required dispersion is realized through propagation in ring-core fiber (RCF) [click], or alternatively with whispering-gallery modes of standard step-index multimode fibers (MMF) [click]. OAM self-imaging on the other hand can be realized with simple azimuthally discretized phase masks, that follow specific Talbot phase relations.
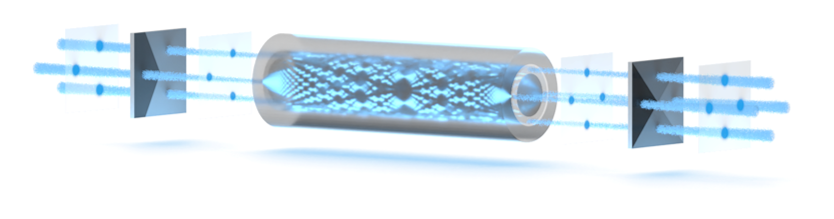
Azimuthal self-imaging alone can be used to realize compact and efficient high-order beamsplitters with just a single short piece of RCF or MMF, where we have shown up to 1-to-30 beamsplitting [click]. Combining the self-imaging effects in angle and OAM leads to a combined tool we call the generalized angle-OAM Talbot effect, with which conversions between fields consisting of different numbers of azimuthal petals (see Fig. 3), or the corresponding OAM combs with different OAM spacings can be performed. We have studied it not only theoretically but also demonstrated it in the lab, and interestingly, the effect can also be used to realize a modulo OAM sorting scheme, capable of theoretically crosstalk-free modulo sorting of OAM modes [click].
Structured Light-Matter Interactions in classical and quantum optics
In addition to studying means to make light more complex and explore its properties, we are also interested in better understanding the interaction of structured light with matter in various aspects.
Linear interaction of structured light with matter
One particularly interesting structure of a light field is our well-beloved phase vortex stemming from azimuthally varying phase that is natural, e.g., to Laguerre-Gauss modes. As the azimuthal order of these modes increases, so does the topological order of its phase singularity. However, under real-world conditions (i.e. in labs) we always have imperfections causing these high-order vortices to split into constellations of unit-charge vortices. Although this sounds like a bug at first, it can be turned into a feature, e.g. when tracking the changes of the constellations after interaction with matter (see Fig. 4). We recently developed a theory describing this change, which we term topological aberration. We showed that we can predict the changes of the vortex constellations also in experiments, which will enable their use to retrieve the properties of the reflective surface, a technique coined as singularimetry [click]. Currently we are exploring new ways to apply our theory to other situations of a dynamical change of vortex constellations.
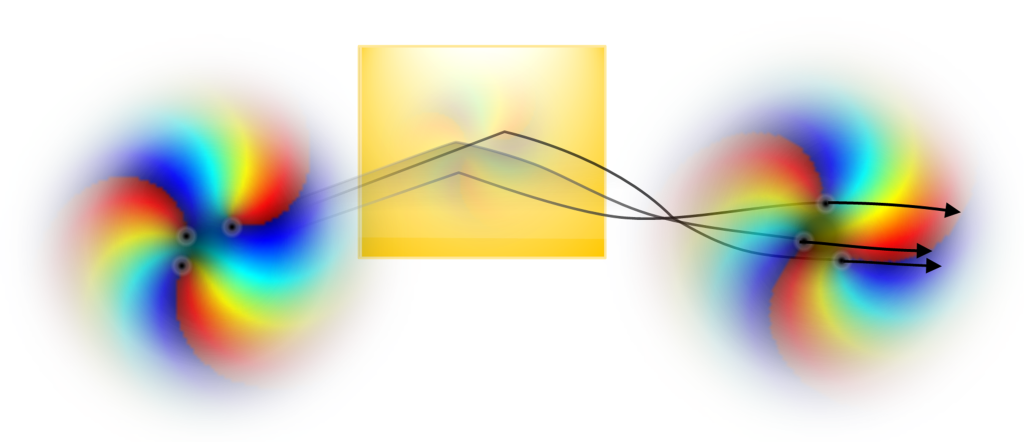
Nonlinear light-matter interactions on the single photon level
As quantum optics researchers, we are also interested in the nonlinear interactions of quantum states of light with matter, of course, with a focus on structured photons (see Fig. 5). In this regard, we work on fundamental ideas, such as the conservation of orbital angular momentum of photons in the nonlinear process of spontaneous parametric down conversion [arXiv]. We also studied, how we can leverage the nonlinear process of sum-frequency generation to build a spin-orbit quantum antenna. Here, we found that it is possible to convert the polarization state (spin) of one photon out of a polarization entangled pair to the OAM state without destroying the entanglement. More interestingly, we were able to show that this conversion only works for a structured laser drive field, which is non-separable in spin and OAM. Hence, we can use the drive field’s classical non-separability to control the quantum non-separability, i.e., entanglement of the photon pair [coming soon].
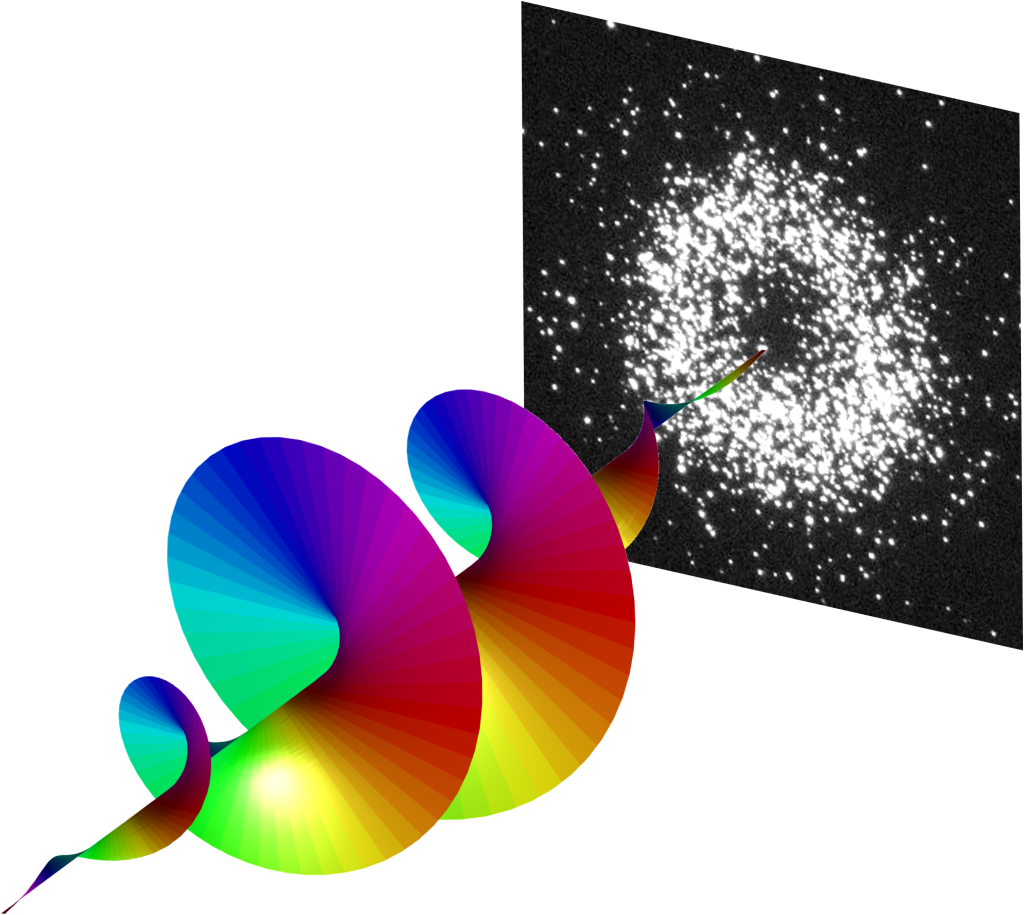
Nonlinear interaction of light with structured matter
While quantum optics and/or structured light is at the focus of most of our studies, we also work (in collaboration with other photonics research groups at Tampere University) on exploring nonlinear optics effects with nano-structured materials. Together with the Nonlinear Optics group, we explore possibilities to increase the efficiency and versatility of metasurface used in nonlinear frequency conversion processes such as second harmonic generation. We were able to show that with a simple open cavity design and multiple transmissions through the sample, the nonlinear signal can be greatly enhanced [click]. With the Nanophotonics group, we demonstrated that the third-order nonlinear Kerr effect can be boosted using guided-mode resonances in a dielectric diffraction grating, such that these silicon nitride samples can be used for optical switching as well as nonlinear pulse shaping [click].
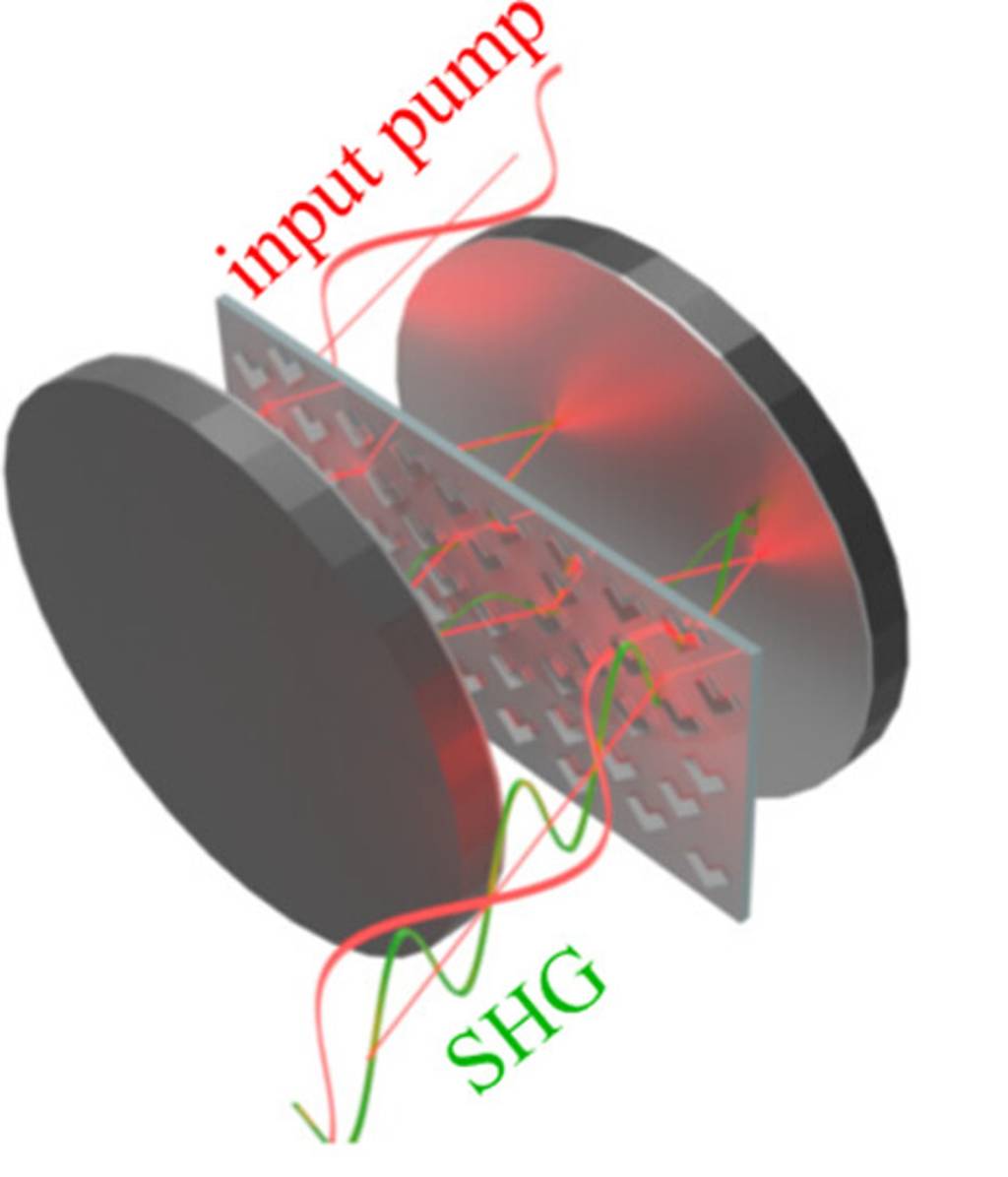
Structured Matter Waves
Finally, the EQO is not only working with lasers and photons, but also single electrons and atoms. In the ERC funded project TWISTION, we study structured matter waves of electron and ions to develop a novel tool for quantum science. Structured matter waves of electrons, atoms, and neutrons have attracted a lot of attention over the last years, as they enable the study of fundamental quantum effects and can lead to novel applications. While electrons are mostly structured in complex transmission electron microscopes setups, we are building our custom-tailored setup to enable the study of yet unexplored energy regimes (~5 keV), enhanced adjustability, novel structures of matter waves, and the possibility to explore novel shaping and measuring mechanisms. In addition, we push towards generating the first structured ion beam to pave the way for novel atom physics research tasks.
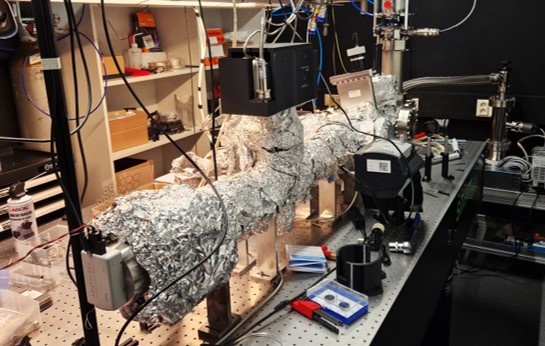
Current and former collaborators
In-house:
> Regina Gumenyuk and her fiber laser group
> Mikko Huttunen and his nonlinear optics group
> Tapio Niemi and his nanophotonics group
> Marco Ornigotti and his theoretical optics group
National:
> Zhipei Sun and his photonics group (Aalto University)
> Andreas Norrman and his quantum optics group (University of Eastern Finland)
International:
> Enno Giese and his group (TU Darmstadt , DE)
> Armin Tavakoli and his group (Lund University, SE)
> Benjamin Stickler and his group (Ulm University, DE)
> Luis Sanchez-Soto and his group (Universidad Complutense de Madrid, ES)
> Marcus Huber and his group (TU Vienna, AT)
> Bob Boyd and his group (University of Ottawa, CA)
> Sven Ramelow and his group (Humboldt University, DE)
> Frederic Bouchard (National Research Council, CA)
> Abuzer Yakaryilmaz (University of Latvia, LV),
> Yuri Chamorovskii and his group (Kotelnikov Institute of Radio Engineering and Electronics, RU)